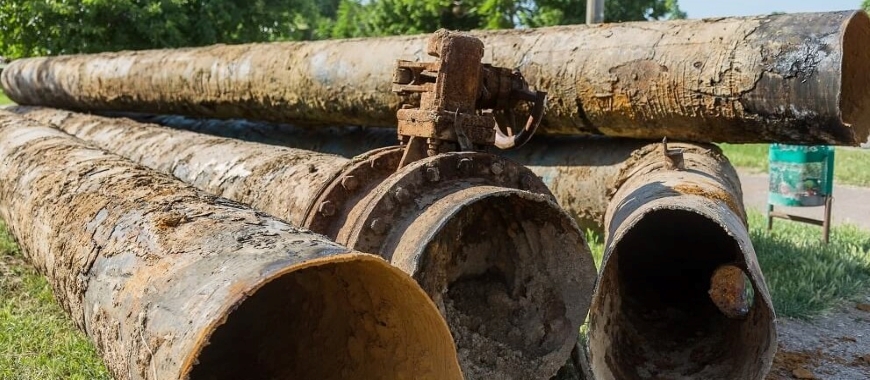
FRP pipe failure modes refer to the various ways in which FRP (Fiberglass Reinforced Plastic) pipes can fail over time due to factors such as mechanical stress, environmental exposure, or improper installation. Understanding these modes is critical for maintaining the structural integrity of FRP piping systems. Common FRP pipe failure modes include delamination, where layers of the pipe separate, cracking due to stress or impact, and fatigue caused by repetitive load cycles. Identifying and addressing these failure modes early can significantly extend the service life of FRP pipes in industrial applications. FRP pipe failure modes include delamination, cracking, fatigue, and joint, corrosion, impact, or bending failures, making installations unusable.
What are FRP Pipe Failure Modes
FRP pipe failure modes encompass various mechanisms by which fiber-reinforced plastic (FRP) pipes can deteriorate or break down under different conditions. Despite the high durability, strength, and corrosion resistance that FRP pipes offer, understanding these failure modes is crucial to ensure their safe and long-lasting application. Failure can occur due to environmental conditions, mechanical stresses, chemical exposure, and manufacturing defects. Identifying and addressing the specific failure modes of FRP pipes provided by GangLong Fiberglass can significantly enhance their performance, reliability, and service life in diverse applications.
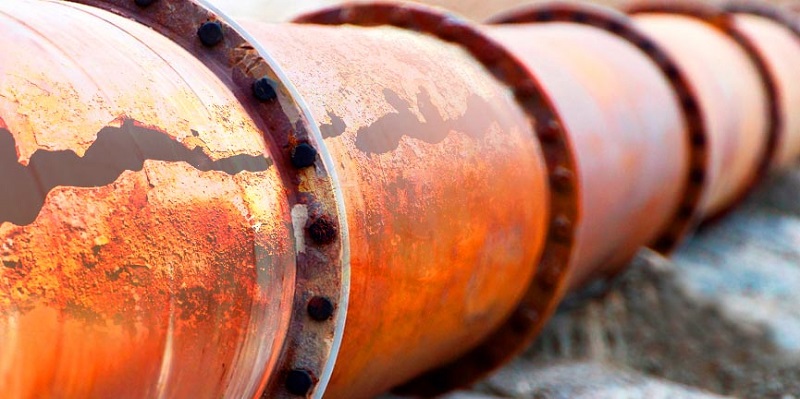
What are the Common Causes of FRP Pipe Failure?
Understanding the common causes of FRP pipe failure is essential for preventing such incidents and ensuring the long-term integrity of piping systems. Various factors contribute to the failure of FRP pipes, and recognizing these causes helps in implementing effective preventive measures.
Mechanical Overload
One of the primary causes of FRP pipe failure is mechanical overload. When FRP pipes are subjected to stresses that exceed their design limits, they can experience structural failure. Mechanical overload can occur due to excessive internal pressure, external loads, or impact forces. For instance, overburden pressure in underground installations or sudden impact from heavy objects can lead to cracks, delamination, or even complete rupture of the pipe.
Chemical Degradation
FRP pipes are designed to resist a wide range of chemicals; however, prolonged exposure to aggressive chemicals, particularly those that are not compatible with the resin matrix, can lead to chemical degradation. This degradation manifests as swelling, softening, or cracking of the pipe material, which compromises its mechanical properties and structural integrity. Chemical degradation is a common failure mode in industrial environments where FRP pipes are exposed to harsh chemicals, solvents, or high-temperature fluids.
Environmental Stress Cracking
Environmental stress cracking (ESC) occurs when FRP pipes are exposed to a combination of mechanical stress and an aggressive environment, leading to the formation of cracks over time. This failure mode is influenced by factors such as temperature, humidity, and chemical exposure. ESC can result in small, surface-level cracks that gradually propagate, eventually compromising the pipe’s structural integrity.
Manufacturing Defects
Defects introduced during the manufacturing process can also contribute to FRP pipe failure. These defects include voids, delaminations, and resin-rich or resin-starved areas within the pipe’s structure. Such imperfections can act as stress concentrators, making the pipe more susceptible to failure under mechanical or thermal loading. Quality control measures during manufacturing, such as proper curing and consistent fiber orientation, are essential to minimize the occurrence of defects.
Fiber Rupture
Fiber rupture occurs when tensile loading is applied close to the fiber axis, exceeding the ultimate tensile strength of the fibers. This failure mode is typically due to improper design or unforeseen excessive loads. Fiber rupture leads to a sudden loss of strength and structural integrity, as the fibers are the primary load-bearing components in FRP pipes.
Buckling
Buckling arises in compression when the loading direction aligns with the fiber axis. The fibers lose their ability to resist compressive forces, causing the pipe to deform or collapse. Buckling is common in thin-walled pipes and is often caused by external pressure or bending loads combined with poor structural support.
Shear Failure
Shear failure occurs at intermediate angles where the load is neither perfectly aligned nor perpendicular to the fiber direction. It results from the matrix’s inability to effectively transfer shear forces between fibers. This failure mode often manifests as delamination or sliding between layers, leading to compromised performance.
Transverse Tensile and Compressive Failures
These failures occur when loads are applied at angles significantly deviating from the fiber axis. The matrix and the fiber-matrix bond bear most of the load in these situations, making them susceptible to cracking, delamination, or crushing. Such failures are particularly common in areas with stress concentrations or defects.
Attachment Joint Failure
Joints in FRP pipes are critical points of stress concentration. Failures can result from improper installation, incompatible materials, or excessive loading at the joints. These failures may involve delamination, cracking, or loss of seal integrity, leading to leaks or structural collapse.
Corrosion-Induced Failure
While FRP is generally resistant to corrosion, prolonged exposure to certain chemicals or environmental factors can degrade the resin matrix. This degradation exposes fibers to environmental damage, weakens the structure, and can lead to delamination or fiber breakage over time.
Temperature-Induced Failure
FRP pipes can fail when exposed to extreme temperatures. High temperatures may soften the resin matrix, reducing its load transfer capability, while low temperatures can make the material brittle. Thermal cycling exacerbates these effects, leading to cracking or delamination.
Impact Failure
Impact damage can result from external forces such as falling objects or collision. These sudden forces can cause localized cracking, fiber fracture, or delamination, often starting as micro-damage that propagates under subsequent loading.
Bend Failure
Excessive bending stresses the outer fibers in tension and the inner fibers in compression. This can lead to fiber rupture, buckling, or matrix cracking. Inadequate support or improper handling during installation often contributes to this failure mode.
The underlying processes that cause these failure modes are called damage mechanisms. The three main damage mechanisms in FRP are:
- Damage to the polymer matrix or resin
- Damage to the reinforcement fibers
- Damage to the interface between the matrix and the fibers
Environmental factors like moisture, UV radiation, and extreme temperatures can also contribute to FRP degradation over time. This can lead to cracking, delamination, or fiber degradation, which can weaken the structural integrity of the FRP.
Fatigue Failure in FRP Pipes
Fatigue failure is a significant concern for FRP pipes subjected to cyclic loading or constant vibration. Over time, the repeated application of stress can weaken the material, leading to the initiation and propagation of small cracks, which can eventually result in catastrophic failure.
Mechanism of Fatigue Failure
Fatigue failure in FRP pipes occurs due to the cyclic nature of loading, such as pressure fluctuations, flow-induced vibrations, or mechanical oscillations. The cyclic stress causes micro-cracks to form within the resin matrix or at the fiber-matrix interface. These micro-cracks act as stress concentrators, leading to further crack propagation with each cycle. As the cracks grow, the material’s ability to withstand stress diminishes, ultimately resulting in failure.
Factors Influencing Fatigue Failure
Several factors influence the fatigue life of FRP pipes and, consequently, the FRP pipe life expectancy, including the magnitude and frequency of the applied stress, the type of resin and fiber used, and the operating environment.Additionally, FRP pipe density plays a significant role, as a higher density can enhance the pipe’s ability to withstand cyclic loading and improve its overall durability in demanding applications.High-frequency cyclic loading and stresses close to the pipe’s ultimate strength significantly reduce fatigue life. The presence of aggressive chemicals, elevated temperatures, and mechanical vibrations can exacerbate fatigue failure, accelerating the crack propagation process.
Detection and Prevention of Fatigue Failure
Early detection of fatigue failure is crucial to prevent catastrophic consequences. Regular inspection and non-destructive testing (NDT) techniques, such as ultrasonic testing and acoustic emission monitoring, can identify the early stages of crack formation in FRP pipes. Preventive measures include optimizing the design to reduce stress concentrations, using high-quality materials from GangLong Fiberglass, and implementing vibration damping systems to minimize cyclic loading. Proper installation practices, such as avoiding sharp bends and ensuring adequate support, can also reduce the likelihood of fatigue failure.
Delamination: A Common FRP Pipe Failure Mode
Delamination is one of the most frequent FRP pipe failure modes, where the bond between the fiberglass layers and resin matrix weakens or separates entirely. This can occur due to various factors such as moisture penetration, improper bonding during the manufacturing process, or prolonged exposure to mechanical stress. When delamination happens, the layers of the FRP pipe start to peel away from one another, significantly reducing the structural integrity of the pipe.
Delamination weakens the overall strength of the pipe, making it more prone to other forms of damage like cracking or bursting. One of the main challenges with delamination is that it may not always be visible on the surface. Often, internal layers of the pipe can start separating while the outer surface appears intact, making regular inspection and advanced diagnostic techniques like non-destructive testing crucial for early detection. In industries using FRP pipes for transporting fluids or chemicals, delamination can lead to leaks, pressure loss, and ultimately, system failure.
Cracking and Fracture in FRP Pipes
Cracking is another major concern when examining FRP pipe failure modes. Cracks in FRP pipes can develop due to excessive mechanical stress, sudden impacts, or even improper installation. Over time, these cracks can propagate through the structure of the pipe, eventually leading to complete fracture or rupture.
Cracking often begins at points of high stress, such as bends, joints, or areas where pipes are subjected to vibration or pressure changes. The nature of FRP materials, which are strong but somewhat brittle, means that once a crack forms, it can spread quickly if the pipe is subjected to continual stress. Cracks can also develop from thermal expansion and contraction cycles, especially in environments where high temperature FRP pipes are exposed to extreme temperature fluctuations. The presence of cracks compromises the pipe’s ability to contain fluids, leading to leaks, pressure losses, and potential environmental hazards.
Proper installation, regular inspections, and controlling the mechanical loads on FRP pipes can help reduce the likelihood of cracking. In industries that use FRP pipes for transporting hazardous chemicals or high-pressure fluids, addressing cracking as part of maintenance routines is critical for ensuring safety and operational reliability.
Creep Failure in FRP Pipes
Creep is another significant mode of failure in FRP pipes, particularly in applications where the pipes are subjected to constant mechanical stress over long periods. Creep occurs when a material slowly deforms under constant load, even if the applied stress is below the material’s failure point. In FRP pipes, creep can cause the pipe to gradually elongate or lose its shape, which weakens the overall structure.
Creep is especially concerning in environments with high temperatures, as heat accelerates the creep process. In industrial settings where FRP pipes are exposed to high-pressure fluids or elevated temperatures, such as steam or chemical processes, creep can significantly reduce the lifespan of the pipe. Over time, the deformation caused by creep may lead to cracks, loss of stiffness, or even rupture.
Laminate Stiffness
Changes in the matrix or resin would be seen as corrosion barrier damage during a traditional visual inspection, but may also affect laminate stiffness. Corrosion barrier damage affects stiffness, usually in direct proportion to the thickness of the corrosion barrier. Inspections have shown that corrosion barrier damage is often detected and quantified before visual inspection.
Sometimes these inspections help to reduce the calculated stiffness values, thus providing conservative values to the owner.
Bonds are commonly used to join FRP structures (secondary bonding). They are used to join shell and pipe sections and for most repairs. Analysis of several types of joints: FRP to FRP joints (e.g. pipe joints), reinforcement of FRP tanks and structures, FRP and carbon fiber applied to steel, and repairs of FRP.
To mitigate creep in FRP pipes, it is essential to use materials that are rated for the specific temperature and load conditions of the application. Choosing the right type of resin and reinforcement fibers, such as those offered by GangLong Fiberglass, can help improve the pipe’s resistance to creep, ensuring that it performs reliably over extended periods.
Effective Adhesive Bonding for FRP Pipe Bell and Spigot Joints
Chemical Degradation of FRP Pipes
Chemical degradation is one of the most dangerous FRP pipe failure modes, particularly in industries where the pipes are exposed to harsh chemicals, acids, or solvents. FRP pipes are generally known for their excellent chemical resistance, but prolonged exposure to aggressive chemicals can eventually weaken the resin matrix that binds the fiberglass reinforcement.
When chemical degradation occurs, the resin starts to break down, compromising the pipe’s strength and structural integrity. This can lead to delamination, cracking, and overall material failure. Additionally, chemical degradation can be accelerated in environments where the FRP pipes are exposed to high temperatures or UV radiation, as these factors further degrade the resin.
To prevent chemical degradation, it is crucial to select the correct resin type for the specific chemicals the FRP pipes will encounter. For instance, GangLong Fiberglass offers a variety of FRP pipe products designed to resist specific chemical environments, ensuring that the pipes maintain their performance over time. Regular inspections and maintenance are also necessary to monitor the condition of the pipes and detect early signs of chemical degradation.
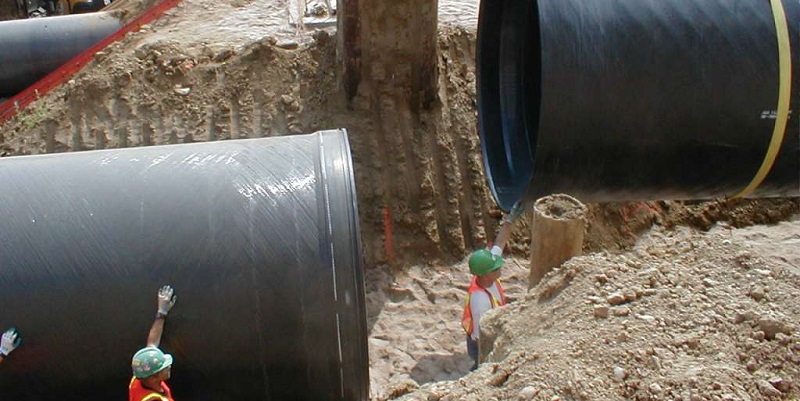
Environmental Factors and FRP Pipe Failure Modes
Environmental factors play a significant role in accelerating FRP pipe failure modes. Exposure to UV radiation, extreme temperatures, and moisture can all contribute to the degradation of FRP pipes over time. For example, prolonged exposure to UV radiation can cause the resin matrix to degrade, leading to surface cracking and weakening of the pipe structure. Similarly, moisture can penetrate the layers of FRP, particularly if there are cracks or delamination, further weakening the bond between the resin and the fiberglass.
In environments where FRP pipes are exposed to extreme temperatures, thermal expansion and contraction cycles can lead to material fatigue, cracking, or even delamination. Understanding how environmental factors impact FRP pipe failure modes is crucial for selecting the right materials and implementing proper protective measures. Using UV-resistant coatings, protective linings, or choosing temperature-tolerant resins can help mitigate the effects of environmental exposure on FRP pipes.
Detecting and Preventing FRP Pipe Failure
Detecting FRP pipe failure modes early is essential for preventing catastrophic failures and extending the lifespan of the piping system. Regular inspections, both visual and through advanced non-destructive testing methods, can help identify early signs of damage such as delamination, cracks, or chemical degradation. Technologies like acoustic emission testing, thermography, or ultrasonic testing can detect internal defects that are not visible to the naked eye.
Preventive maintenance is also key to minimizing the risk of FRP pipe failure modes. Regularly monitoring pressure levels, fluid conditions, and mechanical stress can help identify areas where the pipes may be under excessive load or exposed to corrosive chemicals. Implementing protective measures, such as using corrosion inhibitors, reinforcing high-stress areas, or applying protective coatings, can help reduce the likelihood of failure.
In addition, choosing high-quality FRP pipe products from trusted manufacturers like GangLong Fiberglass ensures that the pipes are made with the right materials and design specifications to handle the intended application. This proactive approach to material selection, combined with regular inspections and maintenance, can significantly reduce the risk of failure and prolong the service life of FRP piping systems.
Best Practices for Installing FRP Pipe Bridge on Industrial Systems
How about Addressing FRP Pipe Failure Modes with Proper Design
Designing FRP piping systems to prevent failure requires a comprehensive approach that considers the material’s unique properties, operating conditions, and potential risks. Below is an expanded discussion on how to address FRP pipe failure modes through proper design.
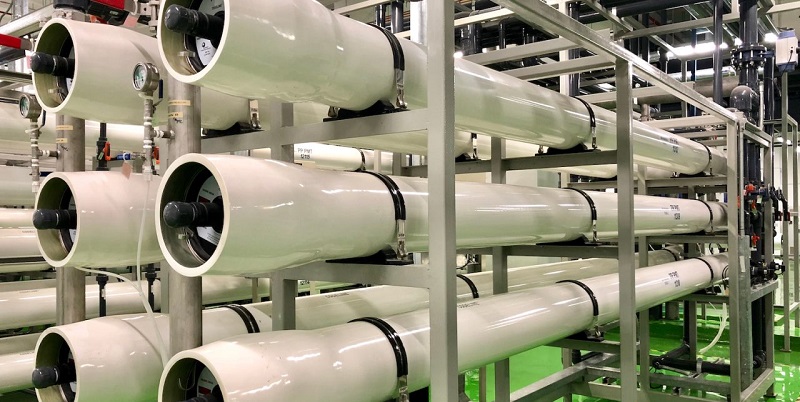
Material Selection
- Fiber Type: Selecting the appropriate fibers (e.g., glass, carbon, or aramid) based on load-bearing requirements is crucial. For instance, carbon fibers provide higher stiffness and fatigue resistance, while glass fibers are cost-effective for moderate loads.
- Resin Type: Choose a resin that suits the chemical and thermal environment. Epoxy resins offer high mechanical strength, while vinyl ester resins are more chemically resistant.
- Additives and Coatings: Incorporating UV inhibitors, fire retardants, or chemical-resistant coatings can enhance durability in specific environments.
Design for Stress Management
- Wall Thickness and Layering: Increase wall thickness or use additional fiber layers in high-stress areas to prevent delamination, cracking, or rupture.
- Reinforcements: Design pipes with localized reinforcements, such as ribs or rings, to handle compressive forces and prevent buckling.
- Load Alignment: Align fibers along anticipated load directions to optimize strength and minimize shear or transverse tensile stresses.
Connection and Joint Design
- Flexible Joints: Use flexible or bellows joints to accommodate thermal expansion and contraction, reducing stress on rigid connections.
- Strengthened Connections: Design attachment points to withstand higher localized stresses, avoiding common failures at joints.
- Seal Integrity: Ensure seals and gaskets are compatible with the pipe material and operational environment to prevent leaks or corrosion.
Thermal Considerations
- Thermal Expansion: Design systems to accommodate expansion and contraction from temperature changes. Flexible joints and support systems can reduce thermal stress.
- Insulation: Use appropriate insulation to minimize thermal cycling effects that could degrade the resin matrix.
Impact and Mechanical Protection
- External Shielding: Protect pipes in high-impact areas with external covers or barriers to reduce the risk of cracks or delamination due to impacts.
- Support Systems: Properly support pipes to prevent excessive bending or localized stress concentrations.
Environmental Adaptations
- UV Protection: Apply UV-resistant coatings or integrate UV inhibitors into the resin to prevent surface degradation from sunlight.
- Chemical Resistance: Consider the long-term effects of chemicals on both the resin and fiber materials, choosing suitable options for aggressive environments.
Inspection and Maintenance
- Regular Inspections: Establish inspection protocols to detect early signs of failure, such as cracking, delamination, or discoloration.
- Non-Destructive Testing (NDT): Use techniques like ultrasonic testing or infrared thermography to assess the pipe’s internal condition without causing damage.
- Proactive Repairs: Address minor damages promptly to prevent the progression of failure.
Standards and Compliance
- Adhere to industry standards such as ISO or ASTM guidelines for FRP design and manufacturing.
- Conduct finite element analysis (FEA) and other simulations during the design phase to predict and mitigate potential failure points.
Education and Training
- Installer Training: Ensure that installers are knowledgeable about handling and joining FRP pipes to avoid damage during installation.
- End-User Awareness: Educate users on operational limits and proper maintenance practices to extend the lifespan of the piping system.
By integrating these principles into the design, FRP piping systems can effectively resist common failure modes, ensuring reliability and safety in even the most demanding environments. Proper design, coupled with material advancements and proactive maintenance, allows FRP pipes to deliver their intended performance over extended service lifetimes.
How to calculate frp pipe friction factor for efficient flow
FAQs about Frp Pipe Failure Modes
FRP (Fiberglass Reinforced Plastic) can experience failure in several ways depending on the application, environmental conditions, and mechanical stress. One common failure mode is delamination, where the layers of fiberglass and resin begin to separate due to poor bonding or prolonged exposure to moisture and environmental factors. This can weaken the structural integrity of the FRP material. Cracking or fracture is another common failure mode, often caused by excessive mechanical loads, improper installation, or physical impact during use.
Additionally, fatigue failure can occur after repetitive stress cycles, particularly in environments where FRP is subjected to constant vibration or load fluctuations. Over time, these small stresses accumulate, leading to eventual failure. Creep failure, where the material deforms gradually under constant load over time, can also occur, especially in high-temperature environments. To minimize these risks, it’s important to follow proper installation practices and use FRP materials within their specified load limits and environmental conditions.
Fiber reinforced composites, like FRP, can fail in several modes, each depending on the load conditions and environmental factors they face. One of the most common failure modes is matrix cracking, which occurs when the resin or matrix material holding the fibers together begins to crack under stress. This can happen when the composite experiences bending, torsion, or tensile loads that exceed its material capacity. Fiber breakage is another failure mode that happens when individual fibers inside the composite fracture due to excessive tensile stress, impacting the overall strength of the composite.
Delamination is a significant failure mode, where the layers within the composite separate due to poor bonding or impact, leading to reduced structural performance. Another failure mode is fiber pullout, where the bond between the fibers and the matrix fails, causing fibers to slide out of the matrix when under stress. Finally, fatigue failure occurs after repeated load cycles, gradually weakening the composite material until it fails. Understanding these failure modes is essential for designing and using fiber-reinforced composites effectively in various applications.
FRP (Fiberglass Reinforced Plastic) can fail through various mechanisms, depending on its application and the environmental conditions it encounters. One common failure mode is delamination, where the bond between the fiberglass layers and the resin weakens, causing them to separate. This usually happens due to moisture penetration, prolonged exposure to high temperatures, or mechanical stress. Cracking is another frequent cause of failure in FRP, especially in areas where the material is subjected to high impact or stress concentrations. Cracks can propagate through the material, compromising its structural integrity.
Another way FRP can fail is through fatigue, where the material gradually weakens due to repeated cyclic loading, such as vibrations or fluctuating forces. Over time, this leads to cracks and eventually causes the material to break. Chemical degradation is also a potential failure mode, especially in environments where FRP is exposed to harsh chemicals that attack the resin, weakening the overall structure. Lastly, creep, or gradual deformation under constant load, can also lead to failure, particularly in high-temperature settings.
Pipelines, including those made of Fiber Reinforced Plastic (FRP), can fail due to various mechanisms:
Corrosion and Chemical Degradation: Exposure to harsh chemicals can weaken FRP’s resin matrix, leading to delamination or surface erosion.
Mechanical Overloading: Excessive pressure, bending, or impact forces can cause cracking, buckling, or rupture.
Thermal Effects: Repeated thermal cycling or exposure to extreme temperatures can degrade FRP’s resin or lead to material expansion and cracking.
Aging and UV Exposure: Over time, environmental exposure can reduce material strength, particularly if UV inhibitors are insufficient.
Manufacturing Defects: Incomplete curing, voids, or inconsistent fiber distribution can act as stress concentrators, initiating cracks under load.
Fatigue: Repeated loading can cause progressive failure, particularly at stress concentrations like joints.
Improper Installation: Misalignment, poor joining techniques, or inadequate support can cause localized stress and premature failure.
Fiberglass, a critical component of FRP, typically fails through these mechanisms:
Delamination: Separation of fiberglass layers due to poor adhesion, excessive shear stress, or resin degradation.
Cracking: Matrix cracking under mechanical or thermal stress exposes fibers to environmental degradation.
Fiber Breakage: High tensile stress or impact can fracture glass fibers, significantly reducing load-carrying capacity.
Moisture Ingress: Water penetration leads to hydrolysis of the resin matrix and loss of mechanical properties.
Stress Concentrations: Defects like voids or scratches amplify stress, leading to localized failure.
Chemical Attack: Harsh environments can degrade the resin and expose fibers, accelerating structural compromise.
UV Damage: Prolonged UV exposure weakens the resin, leading to surface chalking and reduced strength.
Fiberglass, within the FRP context, fails primarily through the interaction of mechanical and environmental stresses:
Matrix Degradation: Heat, chemicals, or UV exposure weaken the resin, which compromises the bond between fibers.
Fiber-Matrix Disbonding: Loss of adhesion between the fibers and resin leads to a reduction in load transfer efficiency.
Mechanical Overload: High stress causes fibers to fracture and the matrix to crack, often starting at defects.
Delamination: Layers separate due to interlaminar shear stress or poor manufacturing quality.
Fatigue: Repeated cyclic loading gradually reduces strength and leads to crack propagation.
Environmental Factors: Moisture, UV, and temperature changes reduce both fiber and matrix performance over time.
Fiberglass fails progressively, often showing micro-cracking or discoloration as early warning signs before catastrophic failure.
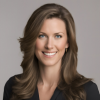
As the editor of GangLong Fiberglass, I have years of experience and in-depth research, focusing on cable tray products, fiberglass solutions, and grille systems. I incorporate years of industry insights and practical experience into every content, committed to promoting the progress of the industry. At GangLong Fiberglass, my commitment is reflected in every product, from innovative cable trays to durable fiberglass solutions and sturdy grille systems. As an authoritative voice in the industry, my goal is to provide valuable information to professionals and businesses and promote forward-looking solutions.