- Home
- Pultrusion Mold
Custom Fiberglass Pultrusion Mold Technology
GangLong Fiberglass design and manufacture of the pultrusion mold are suitable for almost all thermosetting resins used for pultrusion molding.
GangLong Fiberglass stands as a pioneering leader in the field of pultrusion mold, excelling in applications that demand high filler and glass loadings, complex profiles, and high production outputs. Specializing in various pultrusion applications, GangLong Fiberglass enhances manufacturing processes for products such as ladder rails, optical cables, bridge structures, and aerospace components. Our company’s focus on reducing cycle times while maintaining premium quality underscores its commitment to innovation and efficiency in the Pultrusion mold sector.
In addition to its technological prowess in pultrusion, GangLong Fiberglass also offers wholesale pultrusion mold services and mold fabrication for various products, from small, functional items to larger designs like bolection molds used for decorative plating on doors. The Pultrusion mold is vital not only for shaping the composite material but also for ensuring its structural integrity and consistent quality across diverse industries.
Simplifying Your Life: Key Functionalities
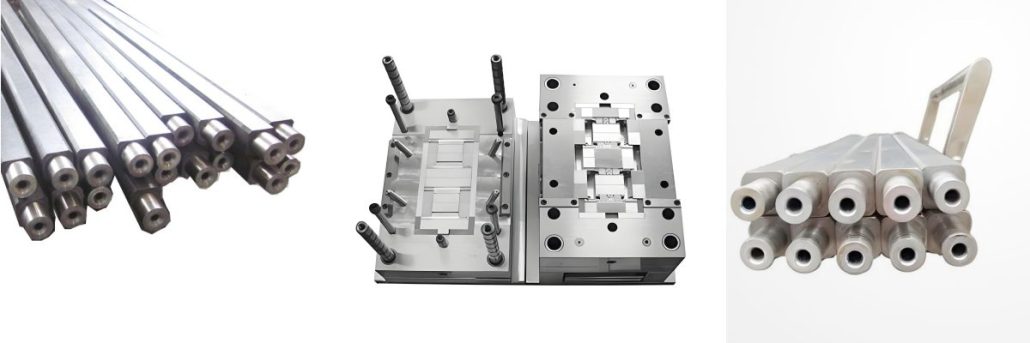
Attribute | Details |
---|---|
Product Name | Pultrusion Mold |
Shaping Mode | Pultrusion Process |
Place of Origin | Hebei, China |
Brand Name | GangLong Fiberglass |
Material | Ortho, ISO, V/E fiberglass, Aluminum Hydroxide (Fire Retardant) |
Surface Treatment | Gritted, Smooth |
Processing Service | Cutting, Custom Service |
Fiberglass Type | E-glass |
UV Inhibitor | UV-9 |
Mold Life | 500,000 - 1,000,000 shots |
Core Components | PLC (Programmable Logic Controller) |
Tolerance | 0.02mm - 0.05mm |
Certifications | ISO 9001 |
Product Application | Walkways, Platforms, Industrial Applications |
After-Sales Service | Technicians available for repair guidance (overseas or via video) |
Warranty | 2 Year |
Surface Finish | Gritted, Smooth, Custom (Polishing, Spray Painting, Screen Printing, etc.) |
Customization | Custom sizes, colors, and surface finishes |
News
- Exploring the Benefits of Carbon Fiber Apparel
- How to Work with Carbon Fiber Successfully
- Carbon Fiber Suit: The Future of Lightweight Armor
- Is Carbon Fiber Armor the Future of Protection?
- Why White Carbon Fiber is Popular in Automotive Design
- Transparent Carbon Fiber Sheets: Strength Meets Clarity in Design
- Chopped Carbon Fiber Mat for Lightweight Reinforcement
- Carbon Fiber EG Hatch: Upgrade Your Civic Today
- The Benefits of Using Structural Carbon Fibre in Engineering
- How Many Layers of Layered Carbon Fiber Are Needed?

Pultrusion Mold Examples
Straight and Bent Profiles
A Pultrusion mold is essential in the manufacturing of both straight and bent profiles, which are widely used in various industries for structural and functional purposes. Pultrusion molds for straight profiles are typically designed to produce long, uniform shapes such as beams, rods, and panels. These profiles are commonly used in construction, aerospace, and automotive industries due to their high strength-to-weight ratio and durability.
In addition to straight profiles, Pultrusion molds can be customized to create bent or curved profiles. These molds are specifically engineered to accommodate the shaping and curing process required to produce curved components, such as arches, angled beams, and custom shapes. These bent profiles are particularly valuable in applications where traditional straight profiles cannot meet design or structural requirements, offering greater flexibility in architectural and engineering projects.
Multi-Cavity Molds
Another significant advancement in pultrusion technology is the development of multi-cavity Pultrusion molds. These molds are designed to produce multiple profiles simultaneously within a single mold setup, significantly enhancing production efficiency. By using a multi-cavity Pultrusion mold, manufacturers can increase output without sacrificing quality, making it an ideal solution for large-scale manufacturing operations.
Multi-cavity molds are especially beneficial in industries that require high volumes of consistent, high-quality profiles, such as the construction of large infrastructure projects or the mass production of automotive components. The ability to produce multiple profiles at once not only reduces production time but also lowers costs, making multi-cavity Pultrusion molds a valuable asset in competitive markets where efficiency and cost-effectiveness are key.
Fiberglass Pultrusion Mold
Fiberglass Pultrusion Mold refers to the specialized mold used in the pultrusion process, which is a continuous manufacturing method for producing fiberglass-reinforced polymer (FRP) composite profiles. These profiles are used in various applications such as construction, automotive, electrical, and aerospace industries due to their high strength-to-weight ratio, corrosion resistance, and electrical insulating properties.
Detailed Description of Fiberglass Pultrusion Mold:
Function and Purpose:
The pultrusion mold is essential in shaping and curing the composite material during the pultrusion process. The mold defines the final shape of the fiberglass profile, allowing it to be pulled continuously from a resin-impregnated fiberglass roving or mat.
Material Composition:
Pultrusion molds are typically made from materials that can withstand high temperatures and mechanical stresses, such as aluminum, steel, or special alloys. These materials are chosen for their durability, thermal conductivity, and resistance to the aggressive resins and curing agents used in the process.
Design Considerations:
- Shape and Geometry: The mold design is crucial for achieving the desired final profile, whether it be simple (such as rods or flat strips) or more complex (such as hollow sections or custom shapes). The mold can have various cross-sectional designs based on the application requirements.
- Ventilation: The mold must have proper ventilation channels to allow the escape of trapped air or gases during the curing process, which helps in preventing defects like bubbles or voids.
- Temperature Control: Molds are typically equipped with heating or cooling systems to control the temperature of the resin during curing. This ensures that the resin polymerizes correctly and that the final product achieves optimal mechanical properties.
- Surface Finish: The surface of the mold is often polished or coated with a release agent to ensure easy demolding of the cured composite parts. A smooth finish is also important for achieving a high-quality surface on the finished product.
The Pultrusion Process:
- Material Feeding: Fiberglass rovings or mats are pulled through a resin bath, where they are impregnated with resin (such as polyester, vinyl ester, or epoxy).
- Molding: After impregnation, the resin-soaked fibers are pulled through the heated mold, where the material is shaped and cured. The mold heats the resin to a specific temperature, triggering the curing process.
- Cooling and Solidification: Once the material exits the mold, it is cooled, solidifying the shape into a rigid, continuous composite profile.
Mold Maintenance:
- Cleaning: Over time, resin residues may build up in the mold, which can affect the quality of the finished product. Regular cleaning and the application of release agents help maintain mold performance.
- Inspection: The mold must be regularly inspected for signs of wear, deformation, or damage that could impact the consistency and accuracy of the pultrusion process.
- Repair: Minor damages to the mold, such as surface wear, can be repaired by resurfacing or re-coating. In more severe cases, sections of the mold may need to be replaced.
Applications of Pultruded Fiberglass Products:
Pultruded fiberglass profiles are used in a wide range of industries:
- Construction: Reinforced beams, columns, and structural components.
- Automotive: Lightweight components for vehicle bodies and structural parts.
- Electrical: Utility poles, electrical enclosures, and components in power generation and distribution.
- Aerospace: Components requiring lightweight, high-strength materials.
The fiberglass pultrusion mold is an integral component in the production of high-quality fiberglass composite profiles. Its design and maintenance are crucial to achieving consistent product performance and ensuring that the final composite structures meet the required strength, durability, and aesthetic standards for various industries. The pultrusion process itself offers significant advantages in terms of efficiency, cost-effectiveness, and the ability to create complex shapes that would be difficult to achieve using traditional methods.
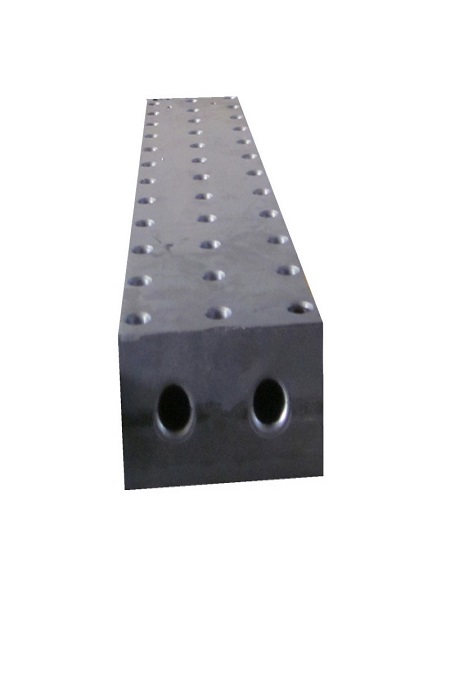

Carbon Fiber Pultrusion Mold
A Carbon Fiber Pultrusion Mold is designed for the production of carbon fiber-reinforced polymer (CFRP) composites using the pultrusion process. Like fiberglass pultrusion molds, they are used to shape and cure continuous profiles of carbon fiber that have been impregnated with resin. The resulting CFRP profiles are known for their exceptional strength-to-weight ratio, stiffness, and resistance to wear, making them ideal for applications in industries like aerospace, automotive, and sports equipment.
Detailed Description of Carbon Fiber Pultrusion Mold:
Function and Purpose:
The mold in carbon fiber pultrusion serves to shape the carbon fiber tow (or fabric) that has been impregnated with resin. As the material is pulled through the mold, it is heated to cure the resin, allowing the composite to harden into a continuous profile of the desired shape.
Material Composition:
Carbon fiber pultrusion molds are typically made from high-strength metals like steel or aluminum, often coated with high-temperature-resistant materials to withstand the stresses and temperatures involved in the curing process. Sometimes, advanced composite materials are also used for mold construction to reduce weight and improve heat dissipation.
Design Considerations:
- Profile Shape: Carbon fiber molds are designed to create a variety of cross-sectional shapes, including beams, rods, tubes, and custom profiles. The mold’s design ensures that the carbon fibers align properly to achieve the desired mechanical properties.
- Temperature Control: Carbon fiber composites require precise temperature control during curing. Molds often feature integrated heating elements or temperature-controlled zones to ensure uniform heat distribution and accurate curing of the resin.
- Ventilation: Proper ventilation is crucial to allow any trapped gases or air to escape during the curing process, preventing voids or air pockets within the carbon fiber composite.
- Surface Finish: The mold surface is carefully finished, often with a polished surface or release agent, to ensure that the carbon fiber part can be easily removed after curing without damaging the profile.
The Pultrusion Process:
- Resin Impregnation: Carbon fiber tows are pulled through a resin bath where they are saturated with resin (such as epoxy or vinyl ester). This step ensures that the fibers are thoroughly coated for optimal bonding.
- Molding and Curing: After impregnation, the resin-saturated fibers are pulled through the mold. The mold heats up, initiating the curing process, which solidifies the composite material into a rigid profile.
- Cooling: Once the material exits the mold, it is cooled, allowing the composite to set fully.
Mold Maintenance:
- Cleaning: Residual resin buildup can negatively impact the mold’s performance, so regular cleaning with appropriate solvents is important to prevent contamination.
- Inspection and Wear: Carbon fiber pultrusion molds are subject to wear, especially at high temperatures. Regular inspection for damage, erosion, or warping is essential to ensure consistent quality of the pultruded profiles.
- Repairs: Minor surface damage can be repaired, but more significant wear may require mold re-coating or replacement of components to maintain mold integrity.
Applications of Carbon Fiber Pultruded Products:
- Aerospace: Lightweight structural components such as beams, frames, and panels.
- Automotive: High-strength parts like chassis components, body panels, and interior elements.
- Sports Equipment: Lightweight, high-performance components for items like bicycles, tennis rackets, and fishing rods.
- Marine: Components requiring both strength and resistance to corrosion, such as masts, hulls, and structural reinforcements.
The Carbon Fiber Pultrusion Mold plays a critical role in shaping and curing CFRP composites, which are used for high-performance applications requiring lightweight yet strong materials. The mold must be engineered to handle the unique properties of carbon fiber, including its tendency to be more brittle than fiberglass and its need for precise temperature control. Overall, the mold’s design, maintenance, and processing steps directly influence the performance and quality of the final carbon fiber product.
Importance of FRP Pultrusion Mold Design
The design of an FRP (Fiberglass Reinforced Polymer) Pultrusion Mold is critical to the success of the pultrusion process and the quality of the final composite product. Pultrusion is a continuous manufacturing method where reinforcing fibers (such as fiberglass, carbon fiber, or aramid) are pulled through a resin bath and then cured in a heated mold to create a solid, rigid profile. The mold design impacts several aspects of the process, including the efficiency, cost, product quality, and consistency. Below, we explore the importance of FRP pultrusion mold design in detail.
Profile Accuracy and Quality
- Shape and Geometry: The mold defines the cross-sectional shape of the pultruded composite part. If the mold is poorly designed, it can result in warped or inaccurate parts, causing issues in downstream applications where precise dimensions are crucial. The mold needs to be engineered to create the exact dimensions and tolerances required by the final product specifications.
- Surface Finish: The quality of the surface finish on the mold directly affects the final appearance of the composite part. A well-designed mold will produce parts with smooth, defect-free surfaces, reducing the need for post-processing like sanding or polishing.
- Fiber Alignment: A good mold design ensures that the reinforcing fibers are properly oriented within the resin matrix, which is essential for achieving the desired mechanical properties, such as strength, stiffness, and impact resistance. Improper fiber alignment can lead to weaker composite parts.
Curing and Thermal Management
- Heat Distribution: The resin in the pultrusion process needs to cure at a specific temperature for optimal material properties. Uneven heating or poor thermal management in the mold can result in incomplete curing, leaving the composite part weak or flexible. A well-designed mold features uniform heat distribution to maintain the required curing temperature and ensure the resin fully polymerizes.
- Cooling Rate: After curing, the composite part needs to be cooled to solidify. The mold design should include features for controlled cooling to avoid warping or internal stresses in the material. Rapid cooling might cause thermal shock, while slow cooling can prolong production cycles.
- Temperature Control Systems: Molds often include integrated heating and cooling systems to regulate the temperature during both curing and cooling stages. A precise control system ensures that the mold temperature stays within an optimal range, enhancing the overall consistency and efficiency of the pultrusion process.
Efficiency and Throughput
- Continuous Production: The pultrusion process is designed for continuous production, which means the mold must allow for uninterrupted operation. Any design flaw that leads to blockages, resin buildup, or other interruptions can slow down or halt production, reducing throughput and increasing downtime. A well-designed mold allows for smooth material flow and consistent output.
- Easy Demolding: A mold that is easy to release from the finished composite part helps minimize production time. Mold release agents, proper cooling mechanisms, and the mold’s surface finish all play a role in ensuring that the composite part can be removed efficiently after curing without causing damage to either the part or the mold.
Mold Durability and Maintenance
- Wear Resistance: FRP pultrusion molds must endure the mechanical and thermal stresses of continuous production. The mold is exposed to high temperatures, friction from the resin-infused fibers, and potential chemical degradation from the resin and curing agents. Therefore, the design must ensure the mold is made from durable materials such as high-strength steels or alloys with wear-resistant coatings to withstand these challenges over time.
- Maintenance and Longevity: Mold design also affects maintenance needs. A well-designed mold should be easy to clean and inspect for wear, with features that reduce the accumulation of resin residue or other contaminants. Regular maintenance of the mold is essential for ensuring its longevity and maintaining consistent product quality.
- Ease of Repair: The mold should be designed with easy access to components that may need to be replaced or repaired, such as heating elements or ventilation systems. Design features that allow quick adjustments or replacements can help minimize downtime during production.
Ventilation and Airflow Management
- Gas Expulsion: During the pultrusion process, air or gases can become trapped within the resin, leading to voids or defects in the final product. Proper ventilation channels in the mold design allow for the escape of these gases, ensuring that the composite material remains free from air pockets. This is especially important for high-performance parts, where voids could compromise the strength and integrity of the composite.
- Optimal Resin Flow: A good mold design promotes uniform resin flow throughout the fiber tow, preventing areas of excess or insufficient resin. This ensures that the fibers are fully impregnated, resulting in a homogeneous composite material that performs consistently.
Cost-Effectiveness
- Material Waste Reduction: Efficient mold design minimizes material waste by ensuring the right amount of resin and fibers are used. Properly designed molds optimize resin flow, reducing excess material that might need to be discarded or recycled. This contributes to overall cost savings, especially in large-scale production runs.
- Cycle Time Reduction: A mold that provides precise control over the curing and cooling processes helps reduce cycle times, enabling higher production throughput. Shorter cycle times lower overall production costs and increase the cost-efficiency of the process.
- Design for Manufacturability: A mold that is easy to manufacture, assemble, and maintain can significantly reduce upfront costs. While complex mold designs may be necessary for intricate parts, simpler, modular designs are often used to lower costs in the initial mold manufacturing phase.
Customization and Adaptability
- Adaptation for Different Applications: The design of the FRP pultrusion mold may vary depending on the type of composite material being used (fiberglass, carbon fiber, aramid, etc.) and the specific application requirements. A well-designed mold can be adapted to produce different shapes or profiles, or it may incorporate modular elements to allow for future customization or adjustments.
- Multifunctional Features: Modern FRP molds may be designed to produce parts with integrated features, such as holes, grooves, or channels. This eliminates the need for secondary operations, improving production speed and reducing costs.
The design of an FRP pultrusion mold is a critical factor that influences the entire production process. A well-designed mold ensures the accurate shaping of composite parts, optimal curing conditions, efficient production, and cost-effectiveness. Additionally, it contributes to the long-term durability and maintenance of the mold, ensuring consistency in quality and reducing downtime. Proper mold design is essential for producing high-performance composite materials used in a variety of industries, from construction to aerospace, and it directly impacts the competitiveness and success of manufacturers in the pultrusion market.


Pultrusion Mold:Preforming mould and Forming mold
In the context of pultrusion, the manufacturing process can involve several types of molds to shape and cure the composite material, and two key stages of molding are typically referred to as the Preforming Mold and the Forming Mold. These molds are integral to the pultrusion process, each serving specific roles in the preparation and shaping of the composite profiles.
Preforming Mold
The Preforming Mold is used in the initial stage of the pultrusion process. Its primary purpose is to prepare the composite material (fiberglass, carbon fiber, or other reinforcements) before it enters the forming mold for final shaping and curing.
Key Functions:
- Fiber Alignment: The preforming mold helps align the continuous fibers (such as rovings or mats) into the correct orientation. This is critical for achieving the desired mechanical properties of the final composite part, such as strength and stiffness. Proper alignment of the fibers ensures that the part can withstand the expected loads and stresses in its end-use application.
- Resin Impregnation: In the preforming stage, the fibers are impregnated with resin (such as polyester, vinyl ester, or epoxy) in a resin bath or by other means. The preforming mold ensures that the resin is evenly distributed over the fibers.
- Initial Shaping: The preforming mold begins to shape the impregnated fibers into a rough or semi-formed profile. This mold doesn’t need to create the final shape of the part, but it starts to provide the basic geometry for the composite. This ensures that the fibers and resin are in the correct alignment and form for the next stage.
- Drying and Consolidation: In some processes, the preforming mold can help consolidate the fibers into a more compact, manageable form before they are pulled into the forming mold. This may also involve partial curing of the resin to make the composite more stable as it moves into the next phase.
Design Considerations:
- The preforming mold is typically designed with features to help maintain fiber tension and prevent any sagging or misalignment of the fibers.
- Ventilation is important to allow air or gases to escape during the fiber alignment and resin impregnation stages, reducing the risk of voids in the final part.
- It is generally designed with a simpler geometry than the forming mold, as its main goal is to prepare the material for the next step rather than finalize its shape.
Forming Mold
The Forming Mold is used in the second stage of the pultrusion process, where the partially formed and resin-impregnated fibers are pulled through and fully shaped into their final profile. After passing through the preforming mold, the composite material enters the forming mold for final shaping, curing, and cooling.
Key Functions:
- Final Shaping: The forming mold is responsible for giving the composite material its final shape. This mold has a precise, detailed cavity that defines the cross-sectional geometry of the composite profile (e.g., beams, rods, tubes, custom shapes). It applies the necessary pressure to ensure that the composite material conforms to the desired shape and density.
- Curing: One of the most important functions of the forming mold is to provide the heat needed for the curing process. The mold is often heated to a specific temperature to initiate and maintain the polymerization of the resin, which hardens and solidifies the composite material.
- Resin Hardening: As the resin impregnated fibers pass through the heated section of the forming mold, the resin undergoes a chemical reaction that causes it to harden, creating a rigid composite part. The precise temperature and time control in the mold ensure that the composite reaches the desired mechanical properties.
- Cooling: After the material exits the curing section of the mold, it needs to be cooled to solidify completely. The forming mold typically includes cooling sections or channels to help control the cooling rate, preventing warping or stresses that might occur if cooling happens too quickly or unevenly.
- Part Extraction: The design of the forming mold includes features to facilitate the easy release of the final part once it has cured and cooled. This can include mold release agents, as well as smooth mold surfaces to prevent any sticking or damage during demolding.
Design Considerations:
- The forming mold is often more complex than the preforming mold, with features like integrated heating elements, temperature control systems, and cooling channels.
- Precision in the mold’s geometry is essential for producing high-quality composite parts with tight tolerances. This is especially important when the parts are used in industries like aerospace, automotive, or construction, where exact dimensions and structural integrity are critical.
- Ventilation and Gas Removal: Proper ventilation is also important in the forming mold to allow for the removal of gases that may form during the resin curing process. Trapped air or gases can create defects in the composite material, such as voids or bubbles.
Both preforming molds and forming molds are crucial in the pultrusion process, but they serve distinct roles. The preforming mold focuses on fiber alignment, resin impregnation, and partial shaping, while the forming mold is responsible for the final shaping, curing, and cooling of the composite material. Each mold type must be carefully designed to ensure optimal fiber orientation, resin impregnation, and curing conditions, which ultimately determine the quality, strength, and precision of the final pultruded composite product. Proper mold design in both stages is essential for achieving high-quality, efficient, and cost-effective production.
The Role of the Pultrusion Operator
Operator Responsibilities
The pultrusion operator plays a crucial role in ensuring the successful execution of the pultrusion process, particularly in the management and operation of the Pultrusion mold. The operator’s responsibilities begin with the setup of the machinery, which includes aligning the Pultrusion mold correctly to ensure the desired shape and dimensions of the final product. This alignment is critical as even minor deviations can result in defects or inconsistencies in the profiles being produced.
Once the setup is complete, the operator is responsible for feeding the raw materials—typically fibers such as glass or carbon—into the machine. This process must be carefully monitored to maintain a consistent feed rate, which directly affects the quality and strength of the composite material. Additionally, the operator must manage the resin bath and ensure that the fibers are adequately impregnated before they enter the Pultrusion mold.
Temperature control is another vital aspect of the operator’s role. The operator must monitor and adjust the temperature of the Pultrusion mold to ensure proper curing of the composite material. If the temperature is too low, the material may not cure properly, leading to weak spots; if it’s too high, it could cause overheating, potentially damaging the mold or the material. Throughout the process, the operator must remain vigilant, making adjustments as necessary to ensure that the profiles produced meet the required specifications and quality standards.
To effectively manage the pultrusion process, an operator must possess a range of technical skills and expertise, particularly concerning the Pultrusion mold and the machinery used in the process. A deep understanding of composite materials is essential, as the operator must know how different fibers and resins interact and how various processing conditions can affect the final product. Technical knowledge of the pultrusion machinery, including the operation and maintenance of the Pultrusion mold, is also crucial. The operator needs to be proficient in using control systems to monitor and adjust parameters such as temperature, pressure, and feed rate. This expertise ensures that the process runs smoothly and that any issues are promptly identified and resolved. Safety is another critical area of focus for the pultrusion operator. Working with high temperatures, chemicals, and moving machinery requires strict adherence to safety protocols to prevent accidents. The operator must also implement quality control measures, such as inspecting the profiles produced and performing regular maintenance on the pultrusion mold and associated pultrusion equipment, to maintain consistent quality and prevent downtime. By ensuring that both the molds and the equipment are in optimal condition, manufacturers can enhance production efficiency and product reliability.Skills and Expertise

Thank you for your interest in our products. To receive our pricelist or for any inquiries, please fill out the form below. We will get back to you within 24 hours.

Fiberglass Pultrusion Machine: Key Components
Machine Overview
A fiberglass pultrusion machine is a complex piece of equipment designed to manufacture continuous composite profiles by pulling fibers through a Pultrusion mold. Pultruded carbon fiber is a high-performance composite material known for its exceptional strength-to-weight ratio, making it ideal for applications where both strength and lightweight characteristics are critical, such as in carbon fiber mesh structures used in various engineering and architectural applications. These fibers are systematically drawn from the creel and guided through a resin bath, where they are thoroughly impregnated with resin, ensuring that the fibers are fully saturated before entering the next stage.
After the fibers are impregnated with resin, they are fed into the heated die, which serves as the Pultrusion mold. The Pultrusion mold is precisely engineered to shape and cure the composite material as it passes through, transforming the resin-soaked fibers into a solid, uniform profile. The mold’s temperature must be carefully controlled to initiate the curing process at the right moment, ensuring the composite material achieves its desired structural properties and dimensional accuracy.
The machine continuously pulls the material through the Pultrusion mold using a powerful haul-off system, which maintains consistent tension and speed. This is critical to producing profiles with consistent cross-sections and high-quality finishes. Finally, the cured composite material exits the mold, where it is cut to the required lengths by an integrated saw or cutting system, ready for further processing or direct application.
A Fiberglass Pultrusion Machine is a specialized industrial machine used to create fiberglass-reinforced polymer (FRP) products. Pultrusion is a continuous manufacturing process that involves pulling fibers through a resin bath and then pulling the resin-saturated fibers through a heated die to create a solid profile. The machine consists of several key components that work together to produce fiberglass composite products such as rods, beams, pipes, and other structural profiles.
Here are the key components of a Fiberglass Pultrusion Machine and their functions:
Fiberglass Reinforcement (Fiber Tow)
- Function: Fiberglass fibers are the primary reinforcement material used in the pultrusion process. The fibers are typically available in the form of continuous rovings, mats, or woven fabrics.
- Material: E-glass or S-glass fibers are commonly used because of their high strength-to-weight ratio and durability.
- Role: The fibers are drawn from supply spools and combined in the proper alignment to provide strength and stiffness to the final product.
Resin Bath/Impregnation System
- Function: The fiberglass reinforcement is passed through a resin bath where the fibers are impregnated with a thermosetting resin, most commonly polyester, vinyl ester, or epoxy resin.
- Resin Flow Control: This system ensures that the right amount of resin is applied to the fibers to achieve the desired mechanical properties and surface finish.
- Importance: Proper impregnation ensures uniformity and high-quality composite products, as uneven resin distribution can lead to weak spots or defects in the final product.
Pulling Mechanism (Puller)
- Function: The puller is a set of rollers or belts that grip and pull the resin-saturated fibers through the entire length of the pultrusion die.
- Role: The pulling mechanism ensures that the fiberglass-resin combination is continuously pulled through the die at a constant speed. This is essential to maintain uniform cross-sections and proper curing of the product.
- Type: Pullers can be mechanical, hydraulic, or pneumatic depending on the machine design.
Heated Die/Die System
- Function: The resin-saturated fibers are pulled through a heated die, where they are shaped into the desired profile (e.g., flat, round, square, or custom shapes).
- Temperature Control: The temperature in the die is crucial to the curing of the resin. It is typically maintained at temperatures of around 150–200°C (302–392°F), depending on the resin type.
- Curing Process: The heat activates the resin’s curing agents, which causes the resin to harden and bond with the fiberglass fibers, forming a solid, rigid composite.
Cooling System
- Function: After the fibers pass through the heated die, the formed composite needs to be cooled to solidify the resin completely and stabilize the material.
- Role: Cooling is usually done via air or water-cooled sections along the extrusion line. The temperature is carefully controlled to avoid warping or distortion of the final profile.
Cutting/Trimming Mechanism
- Function: Once the fiberglass composite exits the cooling system, it is continuously pulled to a length defined by the operator’s settings. The cutting mechanism trims or cuts the continuous profiles to the desired length.
- Types: Cutting can be performed with saws, rotary blades, or other precision cutting tools.
Control System
- Function: The control system of the pultrusion machine manages the operation of all components. It regulates the speed of the puller, the temperature of the die, the resin flow, and other parameters to ensure consistent quality and product specifications.
- Features: Modern pultrusion machines often include PLCs (Programmable Logic Controllers), touch-screen interfaces, and sensors for monitoring temperature, speed, and tension throughout the process.
Coating/Finishing Equipment (Optional)
- Function: Some fiberglass pultrusion systems include additional equipment for coating or finishing the profiles. This may include UV coating for surface protection, painting, or other finishing processes that enhance the appearance or functionality of the final product.
- Role: The finishing equipment ensures the composite products meet specific aesthetic or performance requirements.
Exit Conveyor
- Function: Once the product is formed, cooled, and cut, the exit conveyor moves the finished profiles away from the machine for further processing or packaging.
- Role: It ensures that the finished profiles are safely transported out of the production line and placed in the appropriate staging area for inspection, packaging, or shipment.
Waste Collection System
- Function: During the pultrusion process, there may be waste generated, such as trimmings from the cutting mechanism, off-cuts, or resin spillages.
- Role: A waste collection system collects these by-products for recycling or disposal, minimizing environmental impact and ensuring efficient production.
The fiberglass pultrusion machine is a complex system that combines several key components working in unison to produce high-quality composite materials. The correct integration and functioning of each component are essential for ensuring the final product meets the desired mechanical, thermal, and aesthetic properties. Pultrusion is widely used in industries such as construction, automotive, aerospace, and energy due to the strength, light weight, and corrosion resistance of fiberglass composites.
What is Pultruded Carbon Fiber?
Material Properties
Pultruded carbon fiber is a high-performance composite material known for its exceptional strength-to-weight ratio, making it ideal for applications where both strength and lightweight characteristics are critical. The process of creating pultruded carbon fiber involves pulling continuous carbon fibers through a resin bath and then through a Pultrusion mold. Within the Pultrusion mold, the fibers are precisely aligned and cured to form a rigid, strong profile with consistent cross-sectional dimensions.
Compared to other composite materials, such as fiberglass, pultruded carbon fiber offers superior stiffness and tensile strength, while also being significantly lighter, making it an excellent choice for applications where frp pipe tensile strength is critical. This makes it particularly valuable in industries such as aerospace, where reducing weight without compromising structural integrity is essential. In the automotive sector, pultruded carbon fiber is used to produce components like drive shafts and structural supports that contribute to fuel efficiency and vehicle performance. Additionally, industrial applications benefit from the material’s resistance to corrosion and fatigue, making it suitable for demanding environments.
One of the primary advantages of pultruded carbon fiber is its ability to maintain high strength and stiffness while minimizing weight. This characteristic is especially beneficial in high-performance applications, such as aircraft components, racing car parts, and advanced sporting goods, where every gram of weight savings can translate into significant performance gains. The precision of the Pultrusion mold ensures that the carbon fiber profiles are produced with exacting dimensions and superior surface finishes, which are critical in these demanding applications. However, working with carbon fiber in the pultrusion process also presents several challenges. Handling carbon fibers requires careful attention, as they are more brittle than other fibers, like glass, and can be prone to breakage during the pulling and alignment stages. The curing process within the Pultrusion mold also needs to be meticulously controlled. Carbon fiber’s thermal properties differ from other materials, requiring specific temperature and pressure settings within the mold to achieve optimal curing without compromising the material’s properties. The cost of carbon fiber is higher than that of other fibers, making the pultrusion process more expensive. This necessitates precise control over the process to minimize material waste and ensure that the high-quality output justifies the investment. Despite these challenges, the benefits of using pultruded carbon fiber in applications that demand both strength and lightweight characteristics make it a preferred choice in many advanced manufacturing sectors.Advantages and Challenges


The Pull-Winding Process
The Pull-Winding Process (also known as Pultrusion-Winding or Filament Winding combined with Pultrusion) is a manufacturing process used to create high-performance composite materials, typically for producing parts such as pipes, rods, tanks, and structural elements. It is a hybrid process that combines the pultrusion technique (used for continuous fiber reinforcement) with filament winding (used for precise control over fiber orientation and the creation of hollow or tubular structures). The pull-winding process offers the advantages of both techniques to achieve parts with optimal strength-to-weight ratios, durability, and resistance to harsh environments.
Key Steps in the Pull-Winding Process:
Fiber Reinforcement (Fiber Roving/Tow)
- Function: Continuous fibers (often fiberglass, carbon fiber, or aramid) are pulled from spools to provide reinforcement for the composite material. The fibers are typically in the form of roving or tow (bundles of fibers).
- Material: The most common reinforcement fibers are fiberglass for general applications, or carbon and aramid for specialized high-strength or lightweight applications.
Resin Impregnation
- Function: The fibers are saturated with a thermosetting resin (such as polyester, epoxy, or vinyl ester) to bind them together and create a solid, durable composite once cured. This is typically done using a resin bath or a resin impregnation system, similar to pultrusion.
- Role: The resin saturates the fibers, ensuring they are evenly coated to optimize bonding between the fibers and the resin matrix. The resin also provides the final product with mechanical properties like strength, rigidity, and resistance to environmental factors.
Fiber Winding (Filament Winding)
- Function: After impregnation, the fibers are wound onto a rotating mandrel (or core) in a precise pattern. The winding process involves wrapping the resin-soaked fibers around the mandrel at specific angles and tensions.
- Role: The winding angle (typically helical, hoop, or axial) is controlled to achieve desired mechanical properties in specific directions. This allows the product to have strength in the required load-bearing directions.
- Types of Winding:
- Hoop Winding: Fibers are wound circumferentially around the mandrel, providing strength in the circumferential direction.
- Helical Winding: Fibers are wound at an angle (typically 45°), which provides strength in multiple directions.
- Axial Winding: Fibers are wound parallel to the mandrel axis, providing longitudinal strength.
- Mandrel Types: Depending on the final part, the mandrel can be solid (for solid rods) or hollow (for pipes or tubes).
Pulling Mechanism
- Function: The continuous composite structure is pulled through a curing system after winding. The puller mechanism ensures that the fiber and resin composite is drawn through the entire length of the process at a constant speed, maintaining uniformity and quality.
- Role: The pulling action also helps maintain tension in the fibers, ensuring that the resin-soaked fibers remain well aligned and under the proper tension for optimal strength.
Curing and Heating
- Function: After the fiber winding process, the resin must be cured (hardened) to form the final composite material. Curing is typically achieved by passing the wound material through an oven or heated chamber.
- Role: The heat activates the resin’s curing agents, allowing it to cross-link and solidify. The temperature, time, and resin type are carefully controlled to ensure that the material reaches the desired mechanical properties.
Cooling
- Function: After curing, the composite material must be cooled to prevent warping or distortion. Cooling typically happens as the wound material exits the curing area and enters a cooling section.
- Role: Cooling stabilizes the resin and ensures that the finished composite retains its shape and dimensional accuracy.
Mandrel Removal (For Hollow Parts)
- Function: If the product is tubular or hollow (e.g., pipes), the mandrel used for winding is removed. This can be done either by dissolving a soluble core material or by mechanically extracting the solid mandrel once the composite has cooled and solidified.
- Role: Removing the mandrel allows the final part to be a hollow structure with consistent wall thickness and strength.
Cutting and Finishing
- Function: Once the part has been cured and cooled, it is cut to the desired length and inspected for defects.
- Role: The product can undergo further processes such as trimming, finishing, surface coating, or painting, depending on its application and requirements.
Advantages of the Pull-Winding Process
- High Strength-to-Weight Ratio: The hybrid process combines the strength of continuous fibers with the precise control over fiber orientation, leading to composites with excellent mechanical properties.
- Customization: The fiber winding process allows for precise control over the direction and density of the reinforcement, enabling the creation of custom parts tailored to specific load requirements.
- Versatility: The process can produce both solid and hollow parts, making it suitable for a wide range of applications, including pipes, tanks, rods, and complex structural shapes.
- Durability: The resulting composite materials are often resistant to corrosion, fatigue, and environmental degradation, making them suitable for demanding applications in industries such as aerospace, automotive, and infrastructure.
- Efficiency: The pull-winding process can be highly automated, leading to high production efficiency for continuous or large-scale manufacturing of composite parts.
Applications
The pull-winding process is used in a variety of industries, including:
- Pipes and Tubing: For applications in chemical processing, water transport, and oil and gas.
- Pressure Vessels and Tanks: For industries requiring lightweight, high-strength, and corrosion-resistant containers.
- Structural Components: In aerospace, automotive, and construction industries for lightweight, high-performance parts.
- Marine Applications: Due to its resistance to corrosion, the pull-winding process is used in manufacturing boat hulls and other marine structures.
The Pull-Winding Process is an advanced method for producing high-performance composite materials, combining the benefits of both pultrusion and filament winding techniques. This process enables manufacturers to create strong, lightweight, and durable composite parts with precise control over the fiber orientation and material properties.
Continuous Lamination in Pultrusion
Process Integration
Continuous lamination is a critical process integrated into the pultrusion method to enhance the structural properties of composite materials. In the pultrusion process, fibers are impregnated with resin and then passed through a Pultrusion mold to shape and cure the material. When continuous lamination is applied, additional layers of materials, such as fabrics or mats, are introduced in a continuous manner, bonding with the resin-impregnated fibers as they move through the Pultrusion mold.
This lamination process creates a composite material with multiple layers, each contributing to the overall strength, durability, and flexibility of the final product. The continuous nature of this process ensures that the laminated layers are seamlessly integrated, resulting in a uniform structure without weak points. This is particularly important for applications that require high structural integrity and resistance to environmental factors such as moisture, chemicals, and extreme temperatures.
By incorporating continuous lamination into the pultrusion process, manufacturers can produce composite profiles that are not only stronger but also more adaptable to various uses. The enhanced properties achieved through lamination make these composites ideal for demanding applications in industries such as construction, transportation, and energy.
Examples of Laminated Profiles
Continuous lamination in pultrusion is used to produce a wide range of reinforced composite profiles, each tailored to specific applications. For instance, laminated panels are commonly used in construction for their high strength-to-weight ratio and resistance to weathering. These panels, produced through a Pultrusion mold with continuous lamination, are ideal for building facades, cladding, and structural components that need to withstand harsh environmental conditions while maintaining a lightweight structure.
Another example is laminated decking materials, which are popular in both residential and commercial construction due to their durability and low maintenance requirements. The continuous lamination process allows for the integration of various surface finishes and textures, providing enhanced grip and aesthetic appeal while ensuring long-lasting performance.
In the insulation sector, laminated profiles produced through pultrusion are used to create high-performance insulation materials that offer superior thermal resistance and fire protection. The continuous lamination process ensures that the insulating layers are evenly distributed and securely bonded, resulting in a product that delivers consistent performance across large installations.

Custom Pultrusion Molds for Your Industry Needs
GangLong Fiberglass leverages its manufacturing, engineering, and design expertise to provide custom FRP (Fiber Reinforced Plastic) product solutions tailored to meet the unique needs of its clients. Through advanced processes such as custom pultrusion, open molding, and custom grating, GangLong delivers specialized products for a wide range of industries.
As a custom pultrusion customer, your mold purchase is a one-time cost, and we guarantee that your mold will remain effective throughout the lifecycle of your business. Most profiles utilize cost-effective material combinations, including glass fiber and polyester or vinyl ester resins. We also offer special material options to provide custom-designed stiffness and durability, ensuring your performance requirements are met.
By designing and manufacturing custom pultrusion products in-house, we offer highly competitive pricing alongside high-quality, long-lasting products. Below is a detailed breakdown of the advantages and features of custom pultrusion molds and products:
Advantages of Pultrusion Products
High Strength-to-Weight Ratio: Ideal for lightweight yet strong applications.
Corrosion Resistance: Suitable for harsh environments, including chemical and marine settings.
Heat Resistance: Performs well under high-temperature conditions.
Dielectric Properties: Excellent electrical insulation capabilities.
Dimensional Stability: Maintains shape and size under varying conditions.
Weather Resistance: Withstands UV exposure and extreme weather conditions.
Advantages of Custom Pultrusion Molding
Tailored Designs: Customized to meet the specific needs of your application.
Expert Engineering Support: Assistance from experienced engineers to optimize designs.
Enhanced Strength and Durability: Profiles are optimized for maximum performance and reliability.
Reduced Lifecycle Costs: Durable and efficient designs lower long-term operational expenses.
Key Features of Custom Pultrusion Molds
Pultrusion molds are critical components in the composite production process, determining the dimensional accuracy, quality, and efficiency of FRP profiles. Here’s what sets our custom pultrusion molds apart:
1. Optimal Mold Cavity Design
We accurately estimate the shrinkage rate of pultrusion materials to ensure the final profile dimensions meet your tolerance requirements.
Proper cavity sizing ensures the mold produces profiles that are neither oversized (increasing costs) nor undersized (causing production issues).
2. Expertise in Cross-Sectional Design
Our experience in mold design is derived not only from our extensive design portfolio but also from our hands-on experience in producing FRP profiles for clients.
Unlike other companies, we are both mold manufacturers and users, giving us unique insights into optimizing mold performance.
3. Material Selection
The choice of materials is critical for mold durability and performance. We select high-quality steel types and apply advanced surface treatments to enhance longevity and efficiency.
4. Parting Line Design
We minimize parting lines to ensure tight seams and easy demolding. For seamless molds, this step is unnecessary, further simplifying the process.
5. Versatile Design Options
We offer a wide range of design options for custom molds, including:
Shapes and Sizes: Flat bars, tubes, rods, or complex geometries.
Resin Types: Unsaturated polyester, epoxy, and more.
Fiber Types: Carbon fiber, glass fiber, and others.
Injection Options: Whether or not injection is required.
Seamless Molds: For applications requiring seamless profiles.
Why Choose GangLong Fiberglass for Custom Pultrusion Molds?
End-to-End Solutions: From design to production, we handle every step of the process.
Cost-Effective: Competitive pricing due to in-house manufacturing and design.
High-Quality Standards: ISO-certified processes ensure consistent quality.
Customization: Tailored solutions to meet your specific application needs.
Durability: Molds designed to last throughout your business lifecycle.
GangLong Fiberglass is your trusted partner for custom pultrusion molds and FRP solutions. With our expertise in design, engineering, and manufacturing, we deliver high-quality, durable, and cost-effective products tailored to your unique requirements. Whether you need standard profiles or complex geometries, we have the capabilities to bring your vision to life.
Contact us today to discuss your project and discover how our custom pultrusion molds can enhance your product performance and efficiency!
FAQs about Pultrusion mold
What Are the Disadvantages of Pultrusion?
Another disadvantage is the initial cost of setting up the pultrusion process, particularly the expense associated with creating the custom Pultrusion mold required for specific profiles. This can make the process less cost-effective for low-volume production runs. Additionally, the pultrusion process is less effective with certain types of resins or fibers, particularly those that are difficult to impregnate or cure consistently.
Finally, the mechanical properties of pultruded composites can sometimes be lower in the transverse direction (across the fibers) compared to the longitudinal direction (along the fibers), leading to potential issues with impact resistance or delamination under certain loading conditions. Despite these disadvantages, pultrusion remains a highly valuable manufacturing method for producing composite materials in various industries.
What is Pultrusion in Injection Moulding?
This approach allows manufacturers to take advantage of the high strength and stiffness provided by the pultruded fibers while also benefiting from the complex shapes and surface finishes achievable through injection molding. The resulting composite parts are often lighter, stronger, and more durable than those made using either process alone. This hybrid technique is commonly used in industries such as automotive, aerospace, and consumer goods, where the combination of high-performance materials and intricate design features is required.
What is an example of pultrusion?
What is the mold release agent for pultrusion?
What is the difference between extrusion and pultrusion?
What is the use of pultrusion
What is the principle of pultrusion?
What are the advantages of pultrusion?
What is pultrusion in injection molding?
Which are the elements of pultrusion machine?
Fiber Creel: Holds the spools of reinforcing fibers.
Resin Bath: Where the fibers are impregnated with resin.
Preformer: Guides the resin-impregnated fibers into the correct alignment before entering the die.
Heated Die: Shapes and cures the composite material.
Pulling Mechanism: Pulls the fibers through the entire system.
Cutting System: Cuts the pultruded material into specified lengths.
How does pulforming differ from pultrusion?
These detailed answers can help your customers understand pultrusion and its related processes better. Let me know if you'd like further adjustments!
What is an example of a pultrusion?
What is the advantage of pultrusion process?
High Strength-to-Weight Ratio: Pultruded materials are lightweight yet strong, ideal for applications where weight reduction is important.
Corrosion Resistance: Pultruded composites do not rust or corrode, making them suitable for harsh environments.
Consistency and Efficiency: Pultrusion produces high-quality, uniform profiles at a consistent rate, allowing for mass production with minimal variability.
Low Maintenance: Products made from pultrusion require less upkeep compared to traditional materials like steel or aluminum.
What is an example of a filament fibre?
Which parameter is crucial for controlling the curing process in pultrusion?
What is the difference between pultrusion and pulforming?
Pultrusion is a continuous manufacturing process that produces long, straight profiles with a constant cross-section. Fibers are pulled through a resin bath and heated die, where the composite is cured and shaped.
Pulforming:
Pulforming is similar to pultrusion but allows for the creation of profiles with varying cross-sectional shapes. After pulling the fibers through the resin bath, the composite is molded or shaped into more complex geometries, such as curves or tapered sections.
Key Difference: Pultrusion creates constant cross-sections, while pulforming allows for variable geometries along the length of the part.
What is the use of pultrusion?
Construction: Structural elements like beams, gratings, and handrails.
Telecommunications and Utilities: Utility poles, crossarms, and protective enclosures.
Marine: Corrosion-resistant materials for docks, piers, and marine equipment.
Transportation: Lightweight components for vehicles, boats, and aircraft.
Industrial: Gratings, stair treads, and walkways in corrosive environments.
What are the properties of pultruded fiberglass?
High Strength-to-Weight Ratio: Fiberglass is strong yet lightweight, making it ideal for structural applications.
Corrosion Resistance: Fiberglass resists corrosion from chemicals, moisture, and environmental factors, making it suitable for use in harsh conditions.
Electrical Insulation: Fiberglass is a good electrical insulator, used in electrical utility applications.
Dimensional Stability: Pultruded fiberglass maintains its shape and dimensions under a wide range of temperatures.
Low Maintenance: Pultruded fiberglass products require minimal maintenance compared to metals, as they do not rust or degrade easily.
Is Pulforming similar to pultrusion?
What is the difference between duromers and elastomers?
Duromers (thermosetting plastics) are polymers that undergo an irreversible chemical change during curing. Once cured, they form rigid, strong structures and cannot be reshaped. Examples include epoxy, polyester, and vinyl ester resins.
Elastomers:
Elastomers are flexible, rubber-like polymers that can be stretched and returned to their original shape. They are typically soft, with excellent elasticity. Examples include natural rubber and synthetic rubber materials like silicone or polyurethane.
Key Difference: Duromers are rigid and strong after curing, while elastomers remain flexible and elastic even after curing.
Thank you for your interest in our products. To receive our pricelist or for any inquiries, please fill out the form below. We will get back to you within 24 hours.